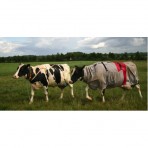
Beyond Reasonable Doubt: The vitamin D piece of the MS puzzle
Clothed cows—and cows wearing only bras—are proving critical in understanding how the body acquires and handles vitamin D and unraveling the vitamin’s role in the development of MS
Last summer, MSDF reported a dramatic study demonstrating that vitamin D supplementation could reverse paralysis in experimental autoimmune encephalomyelitis (EAE) mice and prevent relapse. It’s easy to hope for something similar in human MS patients. Indeed, everyone is waiting for the results of various trials of vitamin D in humans. But those results could be months or years away. The arguments for vitamin D being an effective treatment in MS patients remain tenuous. Yet, as a previous MSDF News Synthesis demonstrated, the evidence for vitamin D deficiency being a major element in the etiology of MS is robust.
The inverse association between serum vitamin D levels and disease severity in MS is undeniable. That and other evidence strongly supports causality. Indeed, Chaplin and Jablonski wrote, “… the contribution of vitamin D deficiency to the manifestation of MS is now beyond reasonable doubt” (Chaplin and Jablonski, 2013).
According to Douglas Goodin’s cascade model of MS (Goodin, 2009), people with a genetic predisposition to MS, low levels of vitamin D, and an exposure to Epstein-Barr virus (EBV) have a high risk of being diagnosed with MS. Using data from Scotland, Ramagopalan et al. (2011) attributed 72% of the variability in MS rates to just two factors: annual spring ultraviolet B (UVB) intensity and infectious mononucleosis (caused by EBV).
In short, vitamin D deficiency substantially contributes to the development of MS, although how much of that contribution happens before and during embryonic development, in childhood, or later is an open question. But once someone is ill, years or decades later, it’s not clear what treating MS with vitamin D can do to reverse disease course. And there are reasons to question the applicability of results in EAE mice to humans. It’s understood that EAE mice do not have the same disease as human MS patients. And while mice may deploy vitamin D in much the same way as humans, mice and other animals may differ in the way they obtain, store, and process vitamin D metabolites.
The evolution of vitamin D metabolism
Many animals such as mice get vitamin D primarily from their food, but humans seem better adapted to synthesizing it from sunlight. Vitamin D forms in human skin when 290- to 315-nm UVB converts a cholesterol-based precursor into previtamin D3. Heat in the skin promotes the conversion of the previtamin into vitamin D3 (cholecalciferol), which circulates in the blood.
According to the vitamin D hypothesis, human skin color has evolved as an adaptational balancing act. Dark skin contains enough melanin to protect circulating folic acid from degradation by UV light and thereby prevents neural tube defects in developing human embryos. Light skin, on the other hand, maximizes vitamin D synthesis in the skin by UV light. Both folic acid and vitamin D are essential to human reproduction.
Vitamin D synthesis is strongly affected by latitude. North or south of about 42 degrees latitude (Boston; Christchurch, NZ), winter sunlight declines sharply, and by 55 degrees (Glasgow; Puerto Toro, Chile) there is not enough annual sunshine to meet the human need for vitamin D. For example, noontime sunshine in central Scotland is insufficient to make any vitamin D for the 8 months between September and May. Scotland is home to many of the most vitamin D–deficient people in the world, but the problem is not limited to Scotland; 91 million people live in cities between 55 and 60 degrees north latitude. Furthermore, 1 billion people worldwide are vitamin D deficient or insufficient.
UVB irradiation decreases more rapidly at higher latitudes, but longer-wavelength UVA (315–400 nm) continues to penetrate the atmosphere at nearly full strength. UVA penetrates more deeply into the skin than UVB does and can reverse vitamin D synthesis in skin samples. “The further north you go,” Penn State’s George Chaplin, M.Sc., explained to MSDF, “the greater the difference between UVA and UVB. The UVB drops off more rapidly as you go north, whereas UVA does not.”
To cope with such low levels of UVB, human populations occupying the highest latitudes have evolved skin as light as human skin can be short of albinism. But even the whitest skin doesn’t provide enough vitamin D in places like Scotland and Norway, so northern populations have met their need for vitamin D by adopting a diet of fish and other high–vitamin D foods.
The polymorphic elements of vitamin D metabolism
Once vitamin D3 from the skin enters the blood, a variety of fates await it. The liver can hydroxylate it to form 25-hydroxyvitamin D3—abbreviated as 25(OH)D3. Vitamin D–binding protein (VDBP) binds 85% to 90% of the circulating serum 25(OH)D3. Almost all of the remaining 25(OH)D3 is bound to albumin (as is a fraction of circulating folic acid). VDBP seems to make the bound vitamin D biologically unavailable, but also increases the vitamin’s half-life in the blood, which is about 15 days in humans (Jones, 2008). So in the absence of sunlight or dietary vitamin D, 25(OH)D3 levels decline over a few weeks.
Virtually any organ in the body can convert available circulating 25(OH)D3 into 1-25(OH)D3—the “active form” of vitamin D3, also called calcitriol. Calcitriol binds the vitamin D receptor (VDR) inside cells. VDR is a nuclear transcription factor that, when bound to vitamin D, alters the expression of genes. In short, VDR mediates the action of vitamin D. VDRs are expressed ubiquitously throughout the CNS, including in both neurons and glial cells. But calcitriol’s half-life is a brief 4 hours (Jones, 2008), and its manufacture is tightly regulated. Vitamin D stored in fat cells may last far longer, but it’s not clear how bioavailable it is.
Many of the molecules involved in vitamin D metabolism are specified by multiple alternate alleles, making each molecular element of the system polymorphic. Because each element is polymorphic, the entire process of vitamin D metabolism can occur in multiple ways depending on the combination of polymorphisms (genetic variants) an individual carries. In addition, the details of vitamin D metabolism in an individual can change in response to environmental or developmental cues. Regardless of which polymorphisms a person carries, the process of handling vitamin D is not necessarily a static trait throughout life but a dynamic (changing) one.
Plants make one form of vitamin D (D2) and animals make another (D3). Organisms produce many polymorphisms of the elements of the vitamin D pathway, including VDBP, VDR, and vitamin D response elements (VDREs). Vitamin D’s synthesis, regulation, and biological effects can differ depending on which variants are present.
VDBP, for example, comes in several forms, with different properties. A person with one VDBP polymorphism might have high levels of bound vitamin D circulating in the blood, whereas someone with another form might have very little. The prevalence of different VDBP polymorphisms can vary by race.
In standard tests of circulating serum 25(OH)D, black Americans display low levels of total 25(OH)D compared to whites, resulting in frequent diagnoses of vitamin D deficiency. Yet, black Americans with supposedly low vitamin D levels frequently have better bone health and higher calcium levels than whites with comparable total vitamin D levels. Polymorphisms in VDBP explain the seeming discrepancy.
According to work by Camille Powe, M.D., of Massachusetts General Hospital in Boston and colleagues (2013), black Americans tend to have low VDBP levels along with their low vitamin D levels. As a result, although they have much lower levels of the bound form of vitamin D, they may have plenty of the VDBP-free bioavailable form and similar, or even higher, levels of 1-25(OH)2D (the active form) than whites.
Ravi Thadhani, M.D., MPH, of Massachusetts General Hospital, a senior author of the Powe paper, spoke with MSDF and speculated that, “What’s bound to the binding protein is not what the cells necessarily see on a routine basis. What’s bound is not necessarily bioavailable. But this is a concept borrowed from research on other hormones.”
Powe and Thadhani found that variation in the VDBP gene explained almost 80% of the variation in VDBP levels. When the effect of genetic variation was controlled, race accounted for less than 0.1% of the variation.
Exactly how the different VDBPs differ remains an open question. Says Thadhani, “We are asking if genotype changes the binding properties of the binding protein—whether it be the half-life, the affinity, the serum levels. Those are things we surmised in our paper, but clearly we need to figure that out more precisely. There’s a huge effort under way to address those specific questions now.”
A plastic, environment-dependent process
Plenty of evidence also supports the idea that the vitamin D system works differently depending on environmental context. There is the migrant effect on MS risk—associated with vitamin D and latitude—in which young people who migrate to a new country carry the MS risk of the country where they grew up. Meanwhile, adult migrants tend to keep the MS risk of their original country. And there’s evidence that the migrant effect can last over multiple generations and that the effect is primarily maternal, suggesting long-term epigenetic effects (Handel et al., 2010). As Oxford neurologist George Ebers, M.D., told MSDF, “There’s evidence that the vitamin D effect goes back through pregnancy and maybe even into a previous generation.”
Likewise, rat pups born to vitamin D–deficient mothers have distinctive alterations in both the gross morphology and microanatomy of their brains, although that effect can be reversed with vitamin D during a critical period later in development.
A striking and influential 2005 BMJ article appeared to show that month of conception strongly influences MS risk, especially at higher latitudes. According to the article, among 42,000 people with MS in northern countries, patients were 9.1% more likely to be born in May and 8.5% less likely to be born in November than people without MS. The study also looked at siblings in which one sibling had MS and the other did not and found that MS patients were 16% less likely to be born in November than their non-MS siblings.
The narrowness of the peak and trough suggest the possibility of a critical period in human development, perhaps at the end of winter and early spring when vitamin D status is lowest. Ebers, an author on the 2005 month-of-birth paper, speculates, “It’s the kind of thing you might see when there’s a threshold effect. Perhaps there’s a very narrow window of time [in early spring] when a person’s vitamin D level dips below a certain threshold.”
But more recent research has argued that these results were a statistical fluke. In a January 2014 paper in the Journal of Neurology, Fiddes et al. assert that by comparing MS month of birth to expected or average seasonal birth rates that don’t control for latitude and year of birth, a false positive is likely. Fiddes et al. specifically object to using averaged national data on latitude and year of birth as the “expected” value. They concluded in their paper, “Many environmental factors are highly heterogeneous within the general population (e.g. smoking and vitamin D levels), raising the possibility that hidden structure could also undermine the testing of these variables.”
Ebers and the paper’s first author, Cristen Willer, don’t agree. In an email to MSDF, Ebers wrote, “Fiddes says that you need to correct for place because place influences birth month. But we went out of our way to do this, using unaffected sib controls, who obviously have the same place in the vast majority of instances. If we leave out those without the same place of birth there is no effect in altering the very high significance. The unaffected sib controls give a larger p value than if general population is used.” The original paper also says, “We found no difference within Canadian patients by sex, site of ascertainment, or decade of birth. Population control results, weighted to match patients with MS for year of birth, were similar to non-weighted controls (not shown).”
Ebers and Willer plan to write a rebuttal to the Fiddes paper. “It is not usual in a rebuttal,” Ebers says, “to be able to point to things the author had overlooked in the original paper.
What’s the right vitamin D metabolite to measure for MS?
Serum 25(OH)D is the easiest vitamin D metabolite to measure. It’s the form normally measured clinically and experimentally, partly because it’s easy to measure, partly because it’s a good predictor of bone pathology. It’s less clear if it’s the best indicator of immune dysregulation.
Lone Hymøller, Ph.D., of Aarhus University in Denmark studies vitamin D in dairy cows. She told MSDF, “The reason 25(OH)D has become the unit of measure is because it’s the form that’s circulating. It’s also [in humans] the storage that we have. We don’t seem to store it very much in organs.” It is difficult to measure 1-25(OH)D, the active form of vitamin D. “1-25(OH)D may be circulating, but not freely. It is very heavily regulated physiologically. Any vitamin D in the body will eventually turn into 25(OH)D, but not necessarily into 1-25(OH)D.”
Thadhani suggests that instead of measuring total circulating serum vitamin D, it might make more sense to measure bioavailable 25(OH)D, the 10% to 15% fraction that isn’t bound to VDBP. But for now there’s no assay for bioavailable vitamin D. “There’s a huge debate about what defines deficiency,” he says. “Our answer is that you can’t define it well unless you have bioavailable levels, but again without an appropriate assay you are left looking at total [25(OH)D] levels.”
And given how much serum vitamin D levels vary in nonpatient populations, the “correct” healthy amount of serum vitamin D is pretty much anyone’s guess.
Cow couture and EAE mice
Vitamin D metabolism is typically presented as a single set of reactions leading inevitably to the active form that binds to a VDR, which in turn alters the expression of hundreds of genes. But the process is highly variable depending on genetics, maternal effects, and exposure to UV light. Mice, rats, and other species all obtain and handle vitamin D in their own way. Dairy cows have serum vitamin D levels similar to those of humans, while horses have almost no circulating vitamin D. Serum vitamin D in Egyptian fruit bats is undetectable, yet the bats are perfectly healthy.
One major way in which animals differ is in whether they obtain vitamin D from sunlight or from food. It’s widely reported that sunlight doesn’t penetrate fur and that, unlike humans, fur-bearing animals, including mice, synthesize vitamin D in their fur and then lick the vitamin off and swallow it.
Hymøller cast doubt on the fur-licking hypothesis when she covered four groups of Holstein dairy cows with different amounts of clothing. Like people, dairy cows make vitamin D only when they are out in the sun and not in the winter months or when they spend their days indoors. In humans, our clothing can block UV light and prevent vitamin D synthesis (Kimlin et al., 2014), and researchers have long assumed that fur does the same. But Hymøller fitted her cows with either no cover, a UV-resistant horse blanket, an udder cover, or the horse blanket and the udder cover. For one summer month, the cows went out in a sunny pasture for 5 hours a day.
As in humans, the more of their body that was covered up, the lower their serum vitamin D levels. Hymøller concluded that fur does not block UVB the way clothing does. Furthermore, because cows lick one another socially, all of the cows should have been able to obtain vitamin D from their uncovered sisters. In that case, vitamin D levels should have been similar in all four groups of cows. Instead, vitamin D levels varied widely by group. Hymøller concluded that cows acquire vitamin D in the same way as most humans, in their skin.
Some animals seem more adapted to getting vitamin D from their food, while others seem adapted to getting it from the sun. Generations of lab mice have obtained their vitamin D from lab chow. Their wild relatives are nocturnal and probably likewise get vitamin D from food. Although mice are capable of synthesizing vitamin D in their skin, it seems unlikely that they get it from their fur if cattle do not.
Vitamin D that comes from food, Hymøller told MSDF, is handled somewhat differently from vitamin D from sunlight. Vitamin D synthesized from sunlight is bound to VDBP, but vitamin D from food travels in the fat fraction of the blood for a while before it is bound to VDBP. “So you have an extra step,” Hymøller says. “There are indications that dietary vitamin D may not be the natural source of vitamin D in humans.”
In short, while it’s useful to study the general biology of vitamin D in MS mouse models, it’s unclear if the details will apply to humans. More to the point, the successful vitamin D treatment of EAE mice may not mean a whole lot for human MS patients. EAE mice develop their version of MS in a very different way, so it’s not a stretch to argue that curing them may involve different processes.
A preventable disease
On the other hand, using our knowledge of MS risk factors to reduce the rate of MS in future generations seems eminently feasible. And vitamin D is one of the strongest contenders.
“If it isn’t vitamin D, what else is it going to be?” Ebers asked. “This is where Bradford Hill was at the end of the 1960s with smoking and lung cancer. If you’re going to be critical, what else could be causing lung cancer if not smoking? What else can it be? Well, it turned out it was smoking. Vitamin D is the same. You can change your risk for MS sixfold by moving from the British Isles to Queensland. What factor other than vitamin D could it be?”
Ebers dismisses UV light by itself as a possible cause of such large migration effects, pointing out that people in northern Norway have half the rate of MS as people in Scotland. Yet they get less sunshine than Scotland’s inhabitants. What they do have is vitamin D—twice the serum vitamin D levels of the Scots, in fact, because they eat foods high in vitamin D. Other factors besides vitamin D also contribute to MS—including exposure to pathogens such as EBV, smoking, and obesity, Ebers said. But all these factors have something in common, he noted. They all have the capacity to induce epigenetic changes. And they are all preventable.
“MS is a preventable disease. Otherwise you wouldn’t be able to change your risk simply by moving.” How to prevent MS is another question. But as Ebers said, “Vitamin D is dirt cheap and it’s harmless, so why not take it? In places where people are really deficient, like Scotland, the government should be thinking about a program for the nation.”
“The thing is, though, that there are things in life that are going to take a long time.” The latency between the recognition of an environmental factor and a disease and the implementation of that knowledge, Ebers says, is about 50 to 75 years. The knowledge that vitamin C could prevent scurvy, that the smallpox vaccine could prevent smallpox, that iodine could prevent goiter and cretinism, or that fluoride could prevent cavities all took decades to implement as public health policy. “Nothing’s changed. It takes just as long now as it did 300 years ago,” Ebers says.
Key open questions
- When in life does vitamin D deficiency have the greatest impact on MS risk—epigenetically in the maternal line, during embryonic development, in childhood, or later?
- What are optimal levels of circulating vitamin D in humans?
- Are measures of bioavailable vitamin D more clinically useful than measuring total serum vitamin D levels?
- How does it happen that the whitest-skinned people—the very people otherwise most highly adapted to ultralow UVB conditions—likewise carry deleterious alleles (for MS) that only affect them under low-UVB conditions?
Disclosures and sources of funding
George Chaplin, M.Sc., reports no disclosures.
George C. Ebers, M.D., serves on the editorial boards of the International Multiple Sclerosis Journal and Multiple Sclerosis and as Section Editor for BMC Medical Genetics; has received funding for travel or speaker honoraria from Bayer Schering Pharma, Sanofi-Aventis, Roche, and UCB; has served as a consultant to Biopartners, Bayer Schering Pharma, Howrey LLP, Heron Health, and Eli Lilly and Company; and receives research support from Bayer Schering Pharma, the Multiple Sclerosis Society of the United Kingdom, and the Multiple Sclerosis Society of Canada Scientific Research Foundation.
Lone Hymøller, Ph.D., reports no disclosures.
Ravi Thadhani, M.D., MPH, reports being a co-inventor on a patent pending on the use of bioavailable vitamin D for the assessment of vitamin D status.