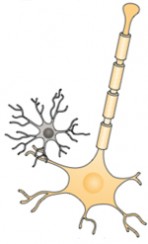
Microglia, Molecularly Defined
Microglia—the innate immune cells of the brain and spinal cord—have long been blamed for stoking MS lesions, but more recent studies suggest they may also have the power to protect tissue and promote repair
In multiple sclerosis (MS), circulating immune cells get plenty of well-deserved bad press for breaching the blood-brain barrier, fanning inflammation, and damaging the myelin sheathing and the underlying axons. But what about the brain’s resident immune cells, known as microglia?
Microglia have long been blamed for stoking MS lesions, but more recent studies suggest they may also have the power to protect tissue and promote repair. A big problem in sorting this out is that activated microglia can be difficult to tell apart from a type of invading immune cell, especially in the inflamed brain tissue common to MS (Biber et al., 2014).
The confusing intruders are known as monocytes when they are traveling in the blood, and they are called macrophages when they migrate into tissue. In a candid acknowledgment of the difficulty in distinguishing these cells under the microscope in the brain, spinal cord, and eye, some studies refer to them as “microglia/macrophages” (Rawji and Yong, 2013).
Now two independent gene expression studies have produced detailed identification kits to distinguish normal microglia from look-alike intruders and from other tissue in normal, aging, and inflamed brains. The telltale molecular cues can help researchers identify microglia with more confidence, dive into the details of microglia’s response to injury and disease, and explore potential therapeutic avenues.
“These will give us better tools to see what’s going on in MS,” said Erik Boddeke, Ph.D., a neurophysiologist at University Medical Center Groningen in The Netherlands, in an interview with MSDF. Boddeke was not involved in either study. Both cell types seem to be active in MS lesions, he said, although they may play different roles in the relapsing-remitting and progressive forms of the disease.
"Why does this matter for MS?" Richard Ransohoff, M.D., a neurologist at the Cleveland Clinic in Ohio, explained to MSDF. "Our treatment strategies are going to be completely different if we think microglia are the problem or if macrophages [are] the problem. Microglia are an important part of the brain. It does not function properly without microglia." Ransohoff, a member of the MSDF Scientific Advisory Board, is a co-author of one of the new studies, and as it happens his nephew, Nathan Kingery, a research technician, is a co-author of the other.
Live long and prosper
Depending on the brain region, microglia represent about 5% to 12% of non-neuronal cells in the central nervous system (CNS). First described nearly a century ago by Spanish neuroscientist Pío del Río Hortega, microglia were thought to arise from bone marrow stem cells, as does most of the immune system.
When activated, microglia and other macrophages morph into amoeba-like blobs. As soldiers of the innate immune system, they are the first essential line of defense against injury and microbes, especially viruses, and are critical in repairing or clearing away damaged tissue. In that role, they consume debris and spew inflammatory molecules, calling in T cells when a stronger immune response is needed (Giunti et al., 2014).
Microglia may be more closely related to macrophages in the skin, spleen, and fat than they are to other brain cells, but there are important differences (Prinz and Priller, 2014). The brain’s innate immune cells live long and seem to replenish themselves behind the blood-brain barrier. Microglia have a complex repertoire of molecular interactions, including a recently discovered crucial role in pruning and remodeling the synaptic connections between neurons. Meanwhile, monocytes seem to charge into the CNS like thugs, generally making trouble.
"In general, microglia are good-natured cells which help the brain and only when things go wrong may turn into an inflammatory cell, which is a lose-lose situation," Boddeke said. Three years ago, he and his colleagues found evidence for a microglia phenotype that supports remyelination amid demyelination, as measured by a gene expression analysis of a mouse model of demyelination (Olah et al., 2011).
"In contrast, [monocytes], by their very nature, are aggressive," Boddeke told MSDF. "They are bad news. If they come in, it means brain damage. If the brain has a choice, it will solve its own problems with its own immune cells in a relatively benign way."
Key differences begin before birth. Several studies in rodents over the past 15 years showed that microglia originate from the yolk sac long before an embryo begins making the blood system (Ginhoux et al., 2013). This newly discovered natural history has created "a new era of microglia research," said Oleg Butovsky, Ph.D., a neuroimmunologist at Brigham and Women’s Hospital (BWH) and Harvard Medical School in Boston, Massachusetts, who led one of the gene expression studies. “The biggest question is, what is the role of microglia in CNS diseases, like MS, Alzheimer's disease, amyotrophic lateral sclerosis (ALS), brain trauma, and glaucoma? Do they help, or do they add some detrimental effect?” The first step was finding a reliable way to study them.
Another major boon to microglia research in the past decade came from meticulous two-photon microscopy movies that show microglia in a live healthy mouse brain, where they appear more like fussy inspectors than aloof sentries. The movies show them busily checking the area around them, extending and retracting mobile processes like so many arms and legs (Nimmerjahn et al., 2005; Hughes, 2012).
Microglia in motion. Several years ago, two-photon microscopic images in healthy mouse brains radically changed the definition of “resting” microglia. Using the same technique, here the green-stained microglia methodically scout their surroundings until a laser makes a pinpoint injury in the mouse cortex. Then, all the armlike sensors quickly converge on the site. Image by Suzanne Hickman, courtesy of Joseph El Khoury.
The newfound ability to single out and study microglia with multiple techniques has implications for a broad swath of neurological diseases. "Without exception, all neuropathological conditions include activation of microglia/monocytes," Butovsky told MSDF. In MS, several lines of evidence suggest that there is a delicate balance between activated microglia being harmful to the myelin-producing cells on one hand, and on the other being necessary for their repair and genesis (Goldmann and Prinz, 2013; Peferoen et al., 2014).
The two recent gene expression studies used different techniques to establish identities for microglia, as distinct from monocytes and other brain cells. "It's not that people didn't know what microglia looked like," said Joseph El Khoury, M.D., a neuroimmunologist at Massachusetts General Hospital in Boston and senior author of one of the new gene expression studies focused on the aging brain. "We just had limited knowledge."
Old mellow microglia
Led by postdoctoral fellow Suzanne Hickman, Ph.D., a team in El Khoury’s lab looked at the impact of aging on the ability of the mouse microglia to sense their environment, with a goal of understanding what may go wrong in Alzheimer’s. They found big differences, including a surprising tendency of the microglia to mellow with age. The microglia of old mice showed a more neuroprotective expression profile compared to those of the younger mice (Hickman et al., 2013). The finding contrasts with other studies suggesting that microglia become more ornery with age, not less. The paper was published online October 27, 2013, in Nature Neuroscience (in print December 2013).
In their study, Hickman and colleagues conducted two major gene expression comparisons in normal adult mice: microglia versus the whole brain, and microglia compared to peripheral macrophages. The team chose direct RNA sequencing to capture an exact count of the transcripts. After sequencing the normal adult mouse microglia, they narrowed the gene expression snapshot to about 1300 potential genes microglia might use to patrol their minipatches of brain. They found a large number of genes in common with macrophages.
Microglia protect against pathogens and support the CNS, but El Khoury and his colleagues wanted to home in on their sensing function. The researchers culled the top 100 molecules that fit three criteria: All were exclusive to microglia, were on the cell surface, and were most likely to detect microbial invasion, inflammatory molecules, and injured cells. They coined a new word to describe these protein-coding transcripts involved in sensing the environment: the "sensome."
To look at the effect of aging, they compared all the transcripts of microglia isolated from mice aged 24 months and 5 months. The team narrowed down their analysis to about 3500 of the most significantly different transcripts and looked for the implicated biological pathways. Unexpectedly, microglia from older mice showed subdued neurotoxic pathways and active neuroprotective pathways.
Drawing on standard definitions of the classic inflammatory macrophage phenotype, called M1, and the more neutral M2 phenotype, the researchers saw slightly more neuroprotective genes expressed in microglia from aged mice compared to those from younger mice, and fewer inflammatory markers overall. Within the smaller sensome set, older microglia sported fewer activated genes known to react to neuronal injury and cell death. El Khoury described these as priming states that may predict how the microglia will react to things they detect in the brain.
“The microglia seem to maintain their ability to fight pathogens but tone down their ability to respond to local endogenous injury,” El Khoury said during a recent lecture at Harvard Medical School. “The microglia in the aging brain are like the perfect politician: tough on defense, but compassionate on social issues.” If microglia ultimately strive for a calm brain environment, he speculated, then it makes sense that they would tone down the genes that respond to neuronal death as the brain ages.
“Now we have a baseline,” El Khoury told MSDF. “The next step is identifying the molecular signatures of microglia, especially what happens to the sensome under different pathological conditions. It’s a work in progress.” The lab is also investigating whether there are different subsets of microglia, how microglia around the brain vary by region, the comparative signature of spinal cord microglia, and how the patterns change in different pathological conditions. Ultimately, for patients, the goal is to harness helpful phenotypes and suppress disease-causing behaviors of microglia, he said.
The new normal
A few miles away, another team in the BWH lab of Howard Weiner, M.D., looked at microglia and other cells from both mouse and human brain tissue in a comprehensive assessment of molecular markers using gene and microRNA arrays and quantitative protein analysis. A slightly different set of just over 100 expressed genes not only distinguished microglia from invading monocytes (called macrophages in tissue) and other immune cells, but also sorted microglia from other cell types in the CNS, including neurons, astrocytes, and oligodendrocytes (Butovsky et al., 2013).
One finding cast doubt on a body of evidence from commonly studied microglial cell lines. They failed to express the newfound signature of adult microglia freshly harvested from the brains of mice and people, as reported by Butovsky and his co-authors. The cell lines could not be salvaged with TGF-β, a molecule they showed was crucial to microglia both in culture and in mice.
Other experiments dashed the notion that monocytes recruited to the brain can replenish microglia. “They do not express the same signature,” Butovsky told MSDF. “They do not function like microglia. It’s that simple. We’re saying that the adult peripheral blood cells don’t give rise to microglia.”
The team also defined a new normal state of microglia, which they and others call M0, in contrast to the classic M1 pro-inflammatory state and the non-inflammatory M2 mode often reported in culture dish studies. “Microglia … are clearly more diverse than an M1 or M2 classification,” they wrote. The paper was published online December 8, 2013, in Nature Neuroscience (in print January 2014).
The study started when Butovsky was seeking a better way to study microglia and their role in disease. It’s not hard to identify microglia in healthy brains. With an intact blood-brain barrier, researchers assume most of the macrophages there are microglia. But all bets were off when it came to inflammatory conditions like MS, where monocytes and microglia mix it up. Researchers have dreamed up elaborate research protocols in mice in ultimately impractical and futile attempts to achieve more precision in tracking down the role of microglia.
For example, in one technique, researchers first irradiated a recipient mouse to ablate the bone marrow and then connected its circulatory system to that of another mouse with labeled monocytes. Those studies seemed to show that monocytes normally infiltrated the brain, replenishing the microglia population.
But then Butovsky and others showed that those results were an experimental artifact caused by radiation damage to the blood-brain barrier. In the corrected parabiotic model with a shielded brain, circulating monocytes could not enter the brain, disabling the dogma that microglia are replenished by blood-borne monocytes. (If the parabiosis technique sounds familiar, it was also the basis of a series of experiments looking for factors in young blood that seem to reverse signs of aging in older mice.)
In the latest study, Butovsky isolated adult microglia and a subset of splenic monocytes known to be recruited to an inflamed CNS from mice. He and his colleagues compared them, first using gene array expression profiles and then using quantitative mass spectrometry to find proteins that were differentially expressed. They culled the list of unique microglial markers and turned to nanostring technology to directly quantify the messenger RNA (mRNA). They constructed specialized mRNA profiling chips for microglia, named MG400. Butovsky presented preliminary findings last year at the American Academy of Neurology meeting (see Meet the Microglia).
In the paper, Butovsky and colleagues report that most of the genes distinguishing microglia from monocytes appear to address their neuronal and developmental functions, in addition to their innate immune activity. The MG400 chip results also detected slight variations in microglia from different parts of the CNS, such as eyes, olfactory bulb, cerebellum, and spinal cord.
The most highly expressed gene in mouse microglia has no human equivalent, but Butovsky and his colleagues found dozens of other genes that separated microglia from immune cells, monocytes, and organ-specific macrophages, including P2ry12, which also turned up in the sensome. Subsequent experiments suggested that the single marker may be sufficient to separate microglia from other immune and brain cells in mice and people.
Meanwhile, Jack Antel of McGill University in Montreal, Canada, had figured out a way to culture human microglia cells extracted from surgical samples. Butovsky learned about Antel’s preliminary work in a phone call the Boston scientist made to share his early gene profiling results. To distinguish the microglia cells from blood-borne monocytes that may have contaminated the samples, Antel found a robust confirmatory signature distinguishing microglia from blood-borne monocytes using the MG400 chip in a blinded experiment.
Reviewers of the paper pushed Butovsky to fully realize his ambitions with a resource that also distinguished the molecular profiles of neurons, oligodendrocytes, and astrocytes. There was also a special request to compare red-pulp macrophages, the spleen cells with the closest molecular signature to that of microglia. He obliged, looping in several more collaborators expert in working with specialized human brain and immune cells.
"We wanted to create something big," Butovsky said. "This will give scientists new tools to investigate microglia. They will be able to generate novel tools like transgenic mice to study microglial cells." For MS, the tools will enable researchers to develop "novel mouse models to manipulate the microglia by specific deletion of genes in microglia or by ablating microglia cells in the CNS without compromising the peripheral immune system," he said. "We identified a tool not just to see microglia but to manipulate microglia in order to understand their biology and their role in homeostasis in disease. This is the major step."
Key open questions
- What are the phenotypes of microglia in different diseases of the CNS?
- How are microglia involved in different stages of MS?
- Can microglia be manipulated therapeutically to treat MS?
Disclosures and sources of funding
Butovsky et al., 2013, was supported by the U.S. National Institutes of Health (NIH), the Amyotrophic Lateral Sclerosis Association, the Department of Defense ALS Research Program, the Thome Foundation, and philanthropic support. Prize4Life provided SOD1 mice. The authors declare no competing interests. Hickman et al., 2013, was supported by the National Institute of Neurological Disorders and Stroke and the National Institute on Aging. The authors declare no competing interests.
Richard Ransohoff is supported by competitive grants from the NIH, National MS Society, Alzheimer's Association, Guthy Jackson Charitable Foundation; by sponsored research agreements with Teva, Genzyme, and Novartis; and by philanthropy from the Williams Family Fund for MS Research.